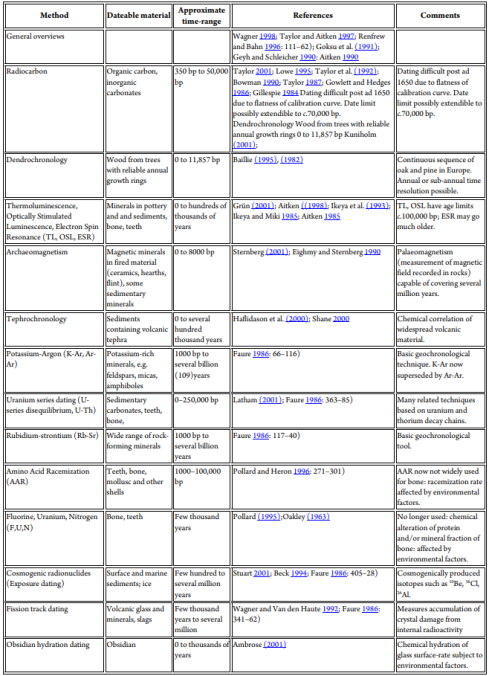
Table 6.1 lists most of the scientific techniques used in archaeology and Quaternary geology, and a guide to some of the relevant literature.
- Radiocarbon
- Dendrochronology
- Thermoluminescence, Optically Stimulated Luminescence, Electron Spin Resonance (TL, OSL, ESR)
- Archaeomagnetism
- Tephrochronology
- Potassium-Argon (K-Ar, ArAr)
- Uranium series dating (Useries disequilibrium, U-Th)
- Rubidium-strontium (Rb-Sr)
- Amino Acid Racemization (AAR)
- Fluorine, Uranium, Nitrogen (F,U,N)
- Cosmogenic radionuclides (Exposure dating)
- Fission track dating
- Obsidian hydration dating
The nature of time
The definition of time used by physicists—for example: ‘The arbitrary choice of a repeatable phenomenon that may be used in the definition of a clock. The choice of the fundamental clock is the definition of time’ (Besançon 1990: 1244)—is functional but spectacularly circular and unsatisfying. In Newtonian time, the repeatable phenomena are astronomical observations, such as the earth circling the sun, or the moon the earth. They do at least provide a practical basis for a calendrical chronology, even if their choice is arbitrary. Even more complex, however, is the relationship between cognition and time. Gould 1991: 10) saw a sharp dichotomy in the perception of time—either as a linear ‘arrow’, or as a never-ending ‘cycle’. In the arrow model: ‘history is an irreversible sequence of unrepeatable events. Each moment occupies its own distinct position in a temporal series, and all moments, considered in proper sequence, tell a story of linked events moving in one direction.’ In the cyclical version: ‘events have no meaning as distinct episodes with causal impact upon a contingent history. Fundamental states are immanent in time, always present and never changing. Apparent motions are parts of repeating cycles, and differences of the past will be realities of the future. Time has no direction.’ Braudel 1980: 3) perceived yet more complexity, considering that historical time occurs at three different ‘wavelengths’. As archaeologists rather than historians, we might struggle with the concept of the longue durée, or of Gould’s never-ending cycles, given our increasing acceptance of the linkage between climate change and human history on even relatively short time-scales (e.g. the ‘little ice age’), and also the potential for sudden cataclysmic events to impinge upon societies’ rhythms (Baillie 1999).
We would, however, recognize social time as the remit of archaeology, although the ‘nervous vibrations’ of individual time might occasionally be sensed in some of archaeological time. Time is, therefore, a difficult concept: ‘time is one of the greatest sources of mystery to mankind’ (Coveney and Highfield 1990: 23). Even more complex is the interpretation of time by different human societies. If history is a cultural construct, then possibly even the passage of time itself is a cultural construct.
Establishing chronologies
Taking Gould’s simple linear portrayal of history as a sequence of events in temporal order, then the most basic requirement of archaeology is the ability to place events in sequential order. This corresponds to creating a relative dating sequence, as defined by O’Brien and Lyman 1999: p.v): ‘a sequence of events for which no fixed or calendric dates exist.’ At the level of the site excavation, this is effected by observing the stratigraphic sequence, on the basic assumption that events evidenced in layers which underlie other layers represent events which precede those above. One of the arts of excavation, of course, is the ability to deal with complex stratigraphies, with intercutting layers, and the ability to identify by cross-correlation contemporary layers which are not physically connected. The principles of stratigraphy were one of the basic concepts in the nineteenth-century revolution in geology, but were proposed in the previous century by James Hutton (1726–97) in his Theory of the Earth (1785). When dealing with archaeological objects (such as pottery, or metal objects) removed from their original stratigraphy, the nineteenth-century model of evolutionary biology gave rise to the concept of seriation, or typological sequences. This is an extension of the everyday observation that artefacts, be they tools, clothing styles, or buildings, change in an observable way over time in a defined geographical (or cultural) region, to the extent that a date can often be guessed from a knowledge of this evolution.
Thus considerable effort was expended in the first half of the previous century in constructing elaborate typologies of many classes of artefact, including pottery, metal weapons, decorative objects, and so on. From these typologies, dates have been assigned to new objects with (sometimes) surprising claims for chronological precision. Both stratigraphy and seriation can only provide a sequence of events or objects—event A occurred before event B, or pottery type C preceded pottery type D. Useful though this is, it does not allow for a precise understanding of when event A occurred, or the time-lapse between pottery types C and D. Up until the 1950s, the tools of stratigraphy and seriation, and their extrapolation via cross-cultural comparisons, provided the only plausible and reasonable mechanisms for establishing chronologies (O’Brien and Lyman 1999). Although some difficulties and challenges were encountered, they provided adequate (and sometimes still useable) frameworks, although the process of cross-cultural comparison, particularly at its geographical extremities, begins to look like a house of cards.
Some issues in radiocarbon dating (Calibration)
It was appreciated very early on in the development of the use of the radioactive decay of carbon-14 as a dating tool that the ‘clock’ needed calibrating in order to bring radiocarbon years into line with calendar years. This was not, by the way, due to any error made by Libby (the main developer of the technique)—it is simply part of the scientific life-cycle process described above. Libby made explicit a number of assumptions when he described the radiocarbon method, including that the rate of production of 14C in the atmosphere and the size of the carbon reservoirs have ‘not changed appreciably’ for ‘20,000 or 30,000 years’ (Libby 1955: 8). If this is so, then the radiocarbon age of a sample is simply calculated (using the law of radioactive decay) from the ratio of the measured 14C activity in the sample to that in a modern sample and a knowledge of the half-life of 14C (estimated by Libby to be 5,568 years). However, like any good scientist, he would have known that any minor variations in these parameters would not invalidate the technique, and he probably recognized that a constant production rate and reservoir size would make the calculations easier, so he adopted these assumptions as a working model. The subsequent demonstration in the early 1970s, by Suess, Clark, and others, using samples taken from dendrochronologically dated wood samples, that radiocarbon ages were not the same as calendar ages over (at least) the last 5,000 years would probably have come as little shock to Libby.
Leave a Reply